There is a direct relationship between the amount of carbon sequestered in soil and CO2 in the atmosphere. The carbon in the soil results from the carbon molecule in CO2 being separated from the O2 molecules and made into sugars and other many complex carbon compounds for use by the plant in its metabolism and growth. For every ton of carbon sequestered in the soil 3.7 tons of carbon dioxide are used (removed) from the atmosphere. Therefore, technologies and natural methods which add increasing levels of carbon to the soil are of critical importance not only because of the CO2 reduction issues but the additional co-benefits of increased soil fertility, food production/security, water absorption and retention, prevention of run off and erosion and many more.
Following is the text of Dr. David Johnson’s important White Paper on carbon sequestration in soil. His field trials using his fungally dominant soil inoculant, BEAM, have demonstrated the incredible potential for the use of his static pile composting method for regenerating soil, and sequestering CO2e at far greater rates than can be accomplished by any other method. This is described in detail below the White Paper.
The Gigacarbon Company has selected Dr. Johnson’s open-source methods to put this approach into effect on an industrial scale, while enriching the Soil Food Web, in combination with Dr. Elaine Ingham’s brilliant prescription for building and maintaining our crucial food production ecosystem.
Dr. Johnson is the Director of the Institute for Sustainable Agriculture at New Mexico State University. At California State University, Chico Campus, he is a Faculty Affiliate and Research Scientist at the Center for Regenerative Agriculture & Resilient Systems; and an Adjunct Professor at the College of Agriculture
“Why Not Soil Carbon? Atmospheric CO2 Reduction in Soils of Agro-ecosystems. A Logical, Practical and Economical Solution!”
Why Not Soil Carbon?
Atmospheric CO2 Reduction in Soils of Agroecosystems- A Logical, Practical and Economical Solution!
In 2009, the U.S. Environmental Protection Agency formally declared carbon dioxide (CO2) a dangerous pollutant, and started looking for: “the most efficient, most economy-wide, least costly, and least disruptive way to deal with CO2 pollution”. There are three approaches for reducing the amount of CO2 in our atmosphere: employ energy efficiency and conservation practices, use low-carbon or carbon-free energy technologies for energy production(renewables), and/or capture and store CO2 from the combustion of fossil fuels or capture it directly from the atmosphere. There have been sustained efforts for significant adoption of conservation and efficiency practices and renewable-energy technologies over the previous two decades; however, global energy use is predicted to increase 25% between now and 2040. Oil consumption will continue to increase in coming decades, due to rising petrochemicals, trucking and aviation demands. Coal, and gas will remain as the largest and second-largest energy resource for electricity generation. Since renewables and conservation practices are not reducing emission of CO2 from fossil fuel use at pace significant enough to reduce atmospheric CO2, the only option remaining is to capture and store the carbon resulting from fossil fuel combustion. The “ideal” attributes for any technology employed to capture and store these carbon emissions are: it must be robust, efficient, safe, economically feasible, and ready to implement. A majority of the currently proposed solutions for capturing and storing atmospheric CO2 do not fulfill any of these attributes.
Carbon Capture Utilization and Storage (CCUS) is promoted as a process for reducing CO2 emissions from power plants, but the economics of CCUS systems are proving to be cost prohibitive. Of the three surviving “pilot” CCUS projects in North America, all have gone over budget and their estimated CAPEX costs are now ranging between $48 to $109 ton-1 of CO2 captured. The add-on costs for: overhead and maintenance ; transportation and storage; parasitic loads; and financing are not included in these estimates, and the cumulative costs for CCUS could more than double the electricity-rate costs for residential and commercial consumers. Besides for the contingencies related to capture, the proof of concept for how to utilize or store the captured CO2 coming from CCUS plants, still remains an issue. Transportation and geo-storage of captured CO2 from a CCUS system carries the potential for migration and leaks, increased seismic activity, and aquifer acidification. The long-term liability issues related to geo-storage will be shouldered by the taxpayer with mechanisms similar to the liability structures in the nuclear energy industry. CCUS projects that employ Enhanced Oil Recovery (EOR) will store no net CO2. Industry estimates for EOR efficiency ranges from 11%-42% of CO2 remaining underground injecting a ton of CO2 into an oil-bearing formation. Other real-world studies estimate between 3.7 and 4.7 metric tons of CO2 are emitted for every metric ton of CO2 injected when considering the amount of oil produced8. Studies concerning readiness and scale have determined that the expected build rates, for future CCUS infrastructures, are now expected to be 100 times too slow and will capture less than 12% of the CO2 reductions needed to meet a 2°C target. Direct-air capture (DAC) using mechanical and/or chemical capture is expected to cost from $92-$234 and potentially up to $600 ton-1 CO2 and storage or utilization is still a problem.
Another alternative CO2 capture and storage technology promotes forest growth on land previously cleared for agriculture. This approach captures small amounts of CO2 as plant biomass. Research on reforestation concluded, in the first 13 years, an average of 200-400 grams dry biomass m-2 year-1 were captured, or a little less than 0.23 tons of carbon captured ha-1 year-1. These carbon sinks are not robust enough and are susceptible to rapid release of captured CO2 if subjected to fire, as witnessed across the Western U.S. the last few years. This approach also has the long-term effect of reducing agricultural land and its potential productivity in a world that is gaining population as it loses productive land. These issues place the expected benefits of cropland re-forestation into uncertainty because cropland has decreased to 0.23 hectares per capita for a world of over 7 billion people. Per capita cropland in 1960 was 0.5 hectares when world population was only 3 billion.13 Another emerging critical agricultural problem, coupled with these land-area reductions, is the degradation in fertility of a significant portion of remaining soils as a result of conventional agricultural practices (plowing, synthetic fertilizer addition, herbicide, insecticide, fungicide applications). Each year 10 million hectares are abandoned due to soil erosion and/or diminished productivity, and another 10 million hectares due to salinization. It is projected that globally, almost one-third of agricultural land has been lost since 1960 and those that remain are losing topsoil at rates considered 6-10 times greater than the rate of soil formation. To compensate, this lost cropland is being replaced by clearing substantial portions of the world’s rain forests promoting land area losses representing more than 60% of the deforestation now occurring worldwide.
As this search for an effective solution continues, concerned governments around the globe are increasing their efforts to reduce CO2 emissions. Countries are making an effort to conform to global treaties designed to reduce global atmospheric CO2 concentrations but almost all are falling short of desired goals.
There is now a viable path forward that will allow robust, practical and economical carbon capture!
The COP 21 officially recognized soil carbon increases as legitimate CO2 offsets on the world stage, opening the door for carbon capture in agro-ecosystems. The EU has created a similar regulatory framework for Climate-Smart Agriculture; however, our conventional approach to agriculture will not be able to participate in this approach as it has been a big part of the problem. Conventional agricultural practices are net emitters of ~6% of annual world CO2 emissions, causing unsustainable losses of soil and biodiversity; and the synthetic fertilizer and pesticide applications used to maintain productivity are polluting the water, air, soils, foods and environment of this planet.
Research at the Institute for Sustainable Agricultural Research (ISAR) at New Mexico State University (NMSU) and California State University Chico’s Regenerative Agricultural Initiative (RAI) is demonstrating a viable, agricultural-based approach to carbon sequestration that avoids the problems related to conventional agriculture while fulfilling the “ideal attributes” for a bio-technological solution to reduce atmospheric CO2 concentrations. This “regenerative” approach to agriculture, if applied at scale, could safely capture and sequester all annual anthropogenic GHG emissions for many decades. It is easy to implement, low cost, scalable, provides multi-decade storage capacity, utilizes a time-tested stable storage medium (soil organic matter), promotes added benefits for improving agro-ecosystem soil fertility and long-term sustainability of our agriculture systems. Adoption in agroecosystems prevents: both near and long-term depletion of natural resources (soil, water, mined nutrients, energy, etc.), soil degradation, biodiversity loss and legacy downstream environmental pollution by avoiding the application of synthetic fertilizers, herbicides, insecticide, and fungicides. This “agricultural approach for carbon capture” is implementable now, with no need to develop new infrastructure or train personnel; it is safe, clean, competitively priced; can be adopted worldwide (third world to first), and will eventually be self-promoting, requiring no further need for financial incentives.
The efficacy of this approach is based on mimicking biological mechanisms that evolved in grassland ecosystems over the last 50+ million years. Grasslands, along with the grazers that frequented them helped develop deep soil carbon profiles by pumping plant exudates through deep-rooted plants along with gradual buildup of high carbon soil surface profiles. This soil carbon build-up was encouraged by the actions of grazing animals (buffalo) herded into tight groups by predators, allowed to only graze about 30%-40% of the available forage, trample down the rest into the topsoil with hoof action and deposit close-proximity dung piles. These dung piles were then visited by dung beetles to roll this dung into balls to be place into the soil for food storage and breeding chambers. The existing soil temperature and humidity allowed this dung to compost in place and produce a soil that had high populations and beneficial structures of microbes. These systems had increased biodiversity and fully functioning soil microbiota that worked mutualistically with the plants. The soils in these systems were re-inoculated with every pass the grazers made through an area. All of these actions stimulated improved forage productivity and significant soil carbon buildup.
ISAR’ and RAI have built research on these biological phenomena, and is observing that promoting beneficial interactions between plants and soil microbes increases farm and rangeland’s efficiency for the capture and storage of carbon in soil. These same interactions produce healthier soils that increase soil microbe carbon-use efficiencies reducing the relative rate at which soil carbon, as CO2, is respired from the soil. When this Biologically Enhanced Agricultural Management (BEAM) technology is promoted in agro-ecosystems, it is feasible to capture and sequester an average of >11 metric tons of CO2 hectare-1 year-1 in rangeland soils21 and >36.7 metric tons CO2 hectare-1 year-1 in transitioning farmland soils for approximately $17-$22 ton-1 CO2, or less than one-tenth the cost of EPA’s recommended Carbon Capture Utilization and Storage (CCUS) technologies.
The health of a soil’s microbiota is crucial for all plant growth occurring on this planet and low carbon stocks within these soil environments are detrimental to soil fertility, microbial community development, and plant survival. Human appropriation of land for agroecosystems has reduced earth’s photosynthetic productive capacity approximately 14.8 Gt C y-1 23 and decreased soil carbon resources 50% to 66% providing a historical carbon loss in soil systems of from 42 to 78 gigatons of soil carbon24. This loss of photosynthetic capacity can be restored through adoption of BEAM management protocols in soils of agroecosystems. The BEAM protocols improve the population, structure, bio-diversity and biological functionality of the microbiota in the soil. This biological functionality includes microbial processes that promote: free-living and symbiotic nitrogen fixation, carbon and nitrogen cycling, elemental (nutrient) metal oxidation, phosphorus solubulization, metabolism of methane and nitrous oxide, antibiotic/antimicrobial production, pesticide/xenobiotics bio-degradation, phytohormone production, and upregulation of biofilm formation andquorum sensing. Re-establishing a diverse and biologically functional microbial community into soil structures enables agricultural systems to mimic the biological dynamics present in natural ecosystems. Natural ecosystems require no nutrient amendments, no tillage, and no weeding, but they remain some of the most productive with regards to biomass production. Old growth forests, riparian zones and estuaries produce the largest terrestrial quantities of annual biomass (1570-1783 grams dry biomass/m2/year)25 and have historically promoted transfer of large quantities of carbon into soils.
ISAR’s research, on BEAM fields in agro-ecosystems, has demonstrated biomass production greater than 3,100 grams dry biomass m-2 year-1, by mimicking nature’s biological barter between plants and microbes using regenerative agriculture practices. This is over double the biomass production of natural ecosystems, and ~3 times greater than the 937 grams dry biomass m-2 year-1, estimated in cultivated land, all from focusing on enhancing microbial communities in agricultural soils.
Slowing the turnover of these carbon components in the soil is a critical issue for the success of this approach. The average mean residence times for organic carbon in boreal, temperate and tropical ecosystems ranges from 200-1200 years. Biomass structure, microbial actions, and environmental conditions are key mechanisms for promoting efficient long-term capture of soil carbon derived from atmospheric CO2. Regenerative agriculture management systems accomplish this by both working with nature to capture larger quantities of atmospheric CO2 through improved growth of biomass, and as well demonstrate increases in soil microbiome carbon-use efficiency with a 4 to 6 times decrease in the relative respiration rate of the carbon. Essentially this approach increases the amount of carbon flowing in” from improved system efficiency for energy (carbon) capture while simultaneously “reducing the amount of carbon flowing out” resulting from microbial community structure related increases in carbon-use-efficiency.
Both the carbon excess in our atmosphere and the carbon deficit in our agricultural soils can be managed with one simple solution: the capture and incorporation of atmospheric CO2 into plant and soil microbial biomass to promote buildup of soil carbon pools, effectively and economically reducing excess atmospheric CO2 to the benefit and promotion of a sustainable regenerative agricultural model.
An electrical utility company could fulfill any commitments it makes for CO2 emissions reduction through purchase of soil carbon offsets from an agricultural trading exchange and offer them to their customers for approximately $0.01/kWh. Fuel costs for gasoline and diesel would realize a ~$0.15 - $0.18 increase per gallon. Airlines could become “Carbon Neutral” where each passenger could contribute less than the cost of a beverage on that flight (~$2.43/passenger for medium distance flights). Each of these examples represents an average 6% increase in energy costs for consumers.
This regenerative approach does not require high-tech equipment or have high costs for implementation. It can be practiced in any country, by farmers and ranchers of any ability, creating economic opportunities and benefits worldwide, even including third world countries. This process treats CO2 as a critical nutrient, not a pollutant, completing a normal, natural cycle for global carbon flow, one that nature has perfected over the last ~437 million years. Atmospheric carbon reduction, resulting from implementation of regenerative agriculture practices, leaves no toxic byproducts, regenerates oxygen back into our atmosphere, builds soil fertility, improves the efficiency of water infiltration, storage and use in agro-ecosystems, while promoting sustainable production of food and fiber.
Due to its low cost, regenerative agriculture will enable and encourage society to proactively address the impacts of increased atmospheric CO2 concentrations while simultaneously easing extraction pressures on natural resources and our environment. Implementation of this agro-ecosystem-based approach, for reducing atmospheric CO2, will buy us time and conserve economic resources that can be better applied towards developing clean energy systems. This approach will offer a CO2 sequestration technology that is traceable, verifiable, reliable, safe, and economically and environmentally friendly. Regenerative agriculture demonstrates a logical solution with multiple benefits for our society, our economy and the environment.
BEAM + Intensive Rotational Grazing
Up to 16 Tons of Carbon Added to Soil / Hectare/Year
Up to 60 Tons of CO2 Removed from the Atmosphere / Hectare/Year
Studies by Dr. Johnson show a 25-times increase in active soil fungal biomass and an annual average capture and storage of 10.27 metric tons soil carbon/ hectare/ year (approximately 38,000 pounds of CO2 /acre/year) Nearly doubles adding ruminants
20-50 times the currently observed soil carbon increase in the 40 equivalent no-till soils tested.
BEAM Benefits
Improves germination and growth rates
Increase crop yields 50-100% or more
Improve water infiltration and retention
Better soil nutrient availability
Increases soil carbon sequestration
Decreased need for fertilizer
Reduces water usage up to 6 times
BEAM + Intensive Rotational Grazing
Up to 16 Tons of Carbon Added to Soil / Hectare/Year
Up to 60 Tons of CO2 Removed from the Atmosphere / Hectare/Year
Studies by Dr. Johnson show a 25-times increase in active soil fungal biomass and an annual average capture and storage of 10.27 metric tons soil carbon/ hectare/ year (approximately 38,000 pounds of CO2 /acre/year) Nearly doubles adding ruminants
20-50 times the currently observed soil carbon increase in the 40 equivalent no-till soils tested.
BEAM Benefits
Improves germination and growth rates
Increase crop yields 50-100% or more
Improve water infiltration and retention
Better soil nutrient availability
Increases soil carbon sequestration
Decreased need for fertilizer
Reduces water usage up to 6 times
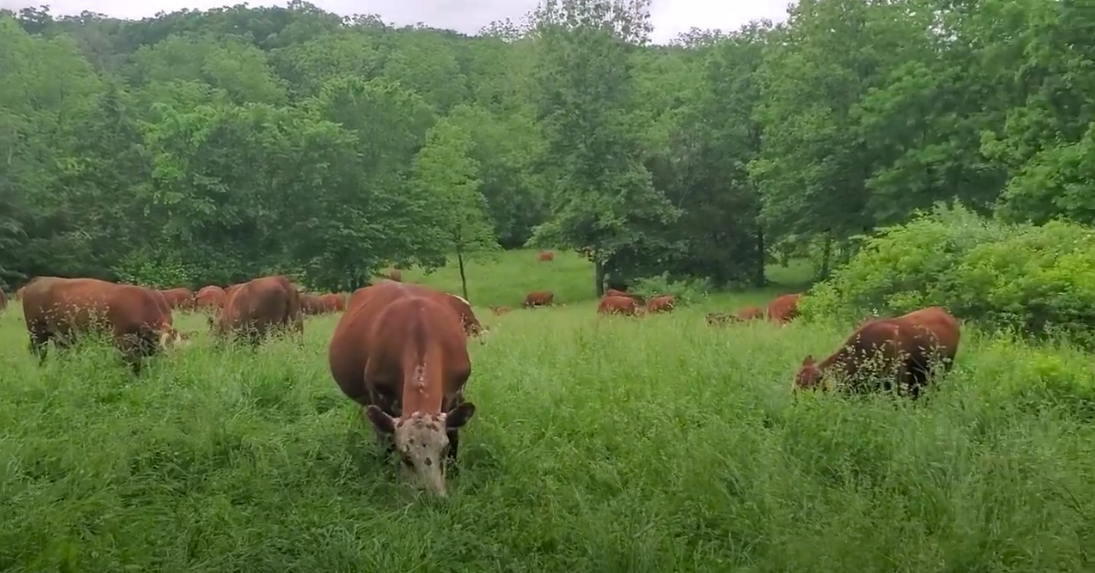
The science and importance of biology for soil health and productivity is gathering momentum. An important center devoted to both research and practical aspects of BEAM has been established at California State University - Chico, California.
Information about this Center (CRA) can be found at the following link.
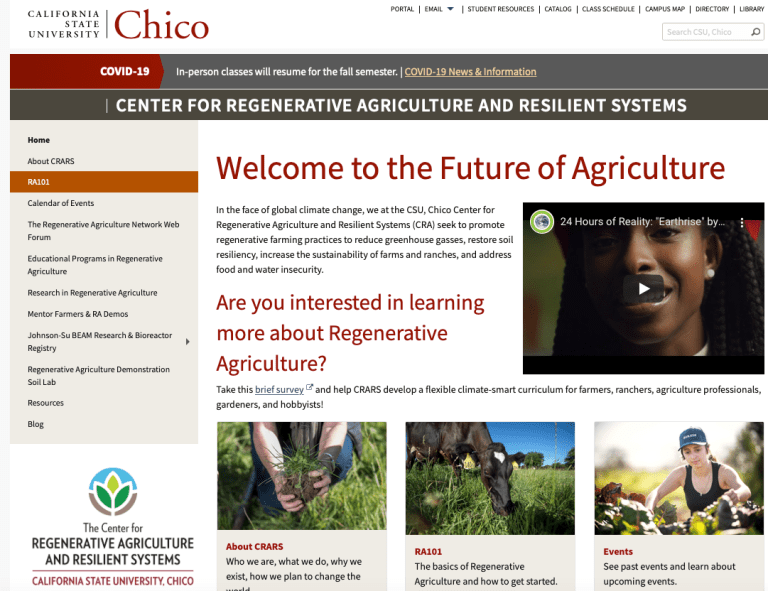
Examples of the wide range of categories of investigation under study are illustrated from their website.